Scope of the Challenge and Menu of Possible Solutions (Synthesis)
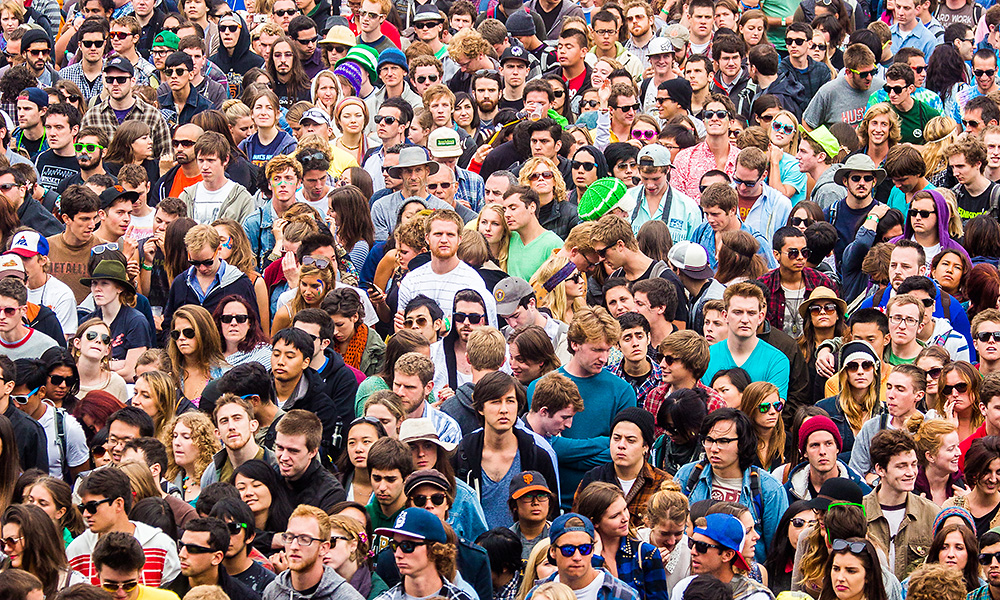
Scope of the Challenge and Menu of Possible Solutions (Synthesis)
-
This World Resources Report addresses a fundamental question: How can the world adequately feed nearly 10 billion people by the year 2050 in ways that help combat poverty, allow the world to meet climate goals, and reduce pressures on the broader environment?
A Recipe for Change
The challenge of creating a sustainable food future involves balancing many competing needs. By 2050, the world must feed many more people, more nutritiously, and ensure that agriculture contributes to poverty reduction through inclusive economic and social development, all while reducing greenhouse gas (GHG) emissions, loss of habitat, freshwater depletion and pollution, and other environmental impacts of farming. Pursuing any one of these goals to the exclusion of the others will likely result in failure to achieve any of them.
We quantify the core of the challenge in terms of the need to close three “gaps”: in food production, agricultural land area, and greenhouse gas (GHG) mitigation. To measure the size of these gaps, we use a new model, GlobAgri-WRR, developed in a partnership between Le Centre de coopération nternationale en recherche agronomique pour le développement (CIRAD), L’Institut national de la recherche agronomique (INRA), World Resources Institute (WRI), and Princeton University (Box 1).
BOX 1 | OVERVIEW OF THE GLOBAGRI-WRR MODEL This global accounting and biophysical model quantifies food production and consumption from national diets and populations, as well as land-use demands. The model also estimates GHG emissions from agriculture, including emissions from production (primarily methane and nitrous oxide), carbon dioxide emissions from the energy used to produce fertilizers and pesticides or to run farm machinery, and emissions from land-use change. Emissions modeled include everything up to the farm gate but do not include those from food processing, transportation, retail, or cooking. GlobAgri-WRR is designed to estimate land use and GHG emissions with specified levels of population, diets and other crop demands, specific trade patterns, and specified agricultural production systems for crops and livestock in different countries. The model by itself does not attempt to analyze what policies and practices will achieve those systems; that is the focus of this synthesis report and the full report. For this reason, GlobAgri-WRR does not attempt to analyze economic feedback effects but concentrates on more biophysical detail. A strength of the GlobAgri-WRR model is that it incorporates other biophysical submodels that estimate GHG emissions or land-use demands in specific agricultural sectors, benefitting from the detail available from other researchers’ work.
The Food Gap
The food gap is the increase above the amount of food (measured as crop calories)1 produced in 2010, the base year for our analysis, to the amount that the world will require in 2050, based on projected demand (Figure 1). Rising food demand over this period—leading to this 56 percent food gap—will be driven by population growth (from 7 billion to 9.8 billion people)2 and by increasing demand for more resource-intensive foods, particularly animal-based foods, as incomes grow.3 Consumption of milk and meat—foods that rely heavily on pasture for their production—is likely to grow by 68 percent. These rates of growth exceed those that prevailed from 1962 to 2010.
The food gap can be closed both through measures that decrease the rate of unnecessary demand growth and measures that increase supply. The more the gap can be closed through demand-reduction measures, the smaller will be the challenge of increasing food production. And as that challenge decreases, so does the risk that the world will fail to meet food needs, which would most harshly affect the poor.
Frequent claims that the world already has an overabundance of food and could meet future needs without producing more food4 are based on an unrealistic, even if desirable, hypothetical. It presumes that the world not only consumes fewer animal products per person, as this report encourages, but by 2050 eliminates nearly all meat consumption; that people shift from meat to vegetables and legumes and consume the same high-yield crops now used for animal feed; that all food loss and waste is eliminated; and that food is distributed just enough and no more than to meet nutritional needs of every person in the world.
Figure 1
The world needs to close a food gap of 56 percent by 2050
Note
Includes all crops intended for direct human consumption, animal feed, industrial uses, seeds, and biofuels.
Source
WRI analysis based on FAO (2017a); UNDESA (2017); and Alexandratos and Bruinsma (2012).
The Land Gap
One strategy to close the food gap could be to clear more land for agriculture—but at the cost of great harm to forests and other ecosystems and the people who depend on them, and large releases of stored carbon from vegetation and soils. Today, croplands and pasture occupy roughly half of all vegetated land.5 Between 1962 and 2010 alone, almost 500 million hectares (Mha) of forests and woody savannas were cleared for agriculture.6 More land clearing would exacerbate a biodiversity crisis driven heavily by land-use change. And virtually all strategies for stabilizing the climate assume no net releases of carbon from land clearing between now and 2050, while many require net reforestation.
Our target is to hold agricultural land area—cropland and pastureland—to the area used in 2010. The land gap is thus the difference between the projected area of land needed to meet global food demand in 2050 and the amount of land in agricultural use in 2010.
The size of the land gap depends on how quickly crop and livestock yields can be improved. If the world were to experience no gains in crop and pasture yields and no moderation in demand for food (what we call our “no productivity gains after 2010” scenario), agricultural land would expand by 3.3 billion hectares, virtually eliminating the world’s forests and savannas. In our baseline projection, we use estimated yields from the Food and Agriculture Organization of the United Nations (FAO), which projects that crop yields will increase, on average, at roughly the same rate as they did between 1961 and 2010. Livestock and pasture productivity gains are from the GlobAgri-WRR model. These gains hold down the expansion of agricultural areas to 593 Mha (Figure 2). However, if future crop yields grow at the somewhat slower rates experienced more recently (1989–2008), and pasture and livestock productivity also grow more slowly than in our baseline scenario, agricultural areas could instead expand by 855 Mha by 2050.
Future yield growth is uncertain, but the key lesson is that the world faces an unprecedented challenge. Crop and pasture yields must increase at rates even faster than those achieved between 1961 and 2010—a period that included the widespread synthetic fertilizer and scientifically bred seeds and a doubling of irrigated area—to fully meet expected food demand and to avoid massive additional clearing of forests and woody savannas.
Figure 2
The world needs to close a land gap of 593 million hectares to avoid further agricultural expansion
Note
“Cropland” increase includes aquaculture ponds.
Source
GlobAgri-WRR model.
The Greenhouse Gas Mitigation Gap
The GHG mitigation gap is the difference between agriculture-related GHG emissions projected for 2050 and an agricultural emissions target for 2050 that is necessary to help stabilize the climate at globally agreed targets.7
Agriculture and land-use change contributed one-quarter of total human-caused GHG emissions in 2010—roughly 12 gigatons (Gt) measured as carbon dioxide equivalent (CO2e).8 Of this total, a little more than half resulted from agricultural production, including such sources as methane from livestock production and rice cultivation, nitrous oxide from nitrogen fertilizer, and carbon dioxide released by fossil fuels used in agricultural production.9 A little less than half of the emissions resulted from land-use change (vegetation clearing and soil plowing) as agriculture expanded. The land-use category includes 1.1 Gt released annually by the ongoing degradation of cleared peatlands, which are carbon-rich soils that decompose and sometimes catch fire once drained for agriculture.10
Using the GlobAgri-WRR model, we project total agricultural GHGs to be roughly 15 Gt per year in 2050–9 Gt of annual emissions from agricultural production and an annual average of 6 Gt between 2010 and 2050 from agricultural expansion and drained peatlands.11 What are the implications of this estimate? Modeled strategies for holding climate warming to the global target of 2 degrees Celsius (2°C) (3.6 degrees Fahrenheit) above preindustrial levels typically require that total emissions from all human sources in 2050 amount to no more than around 21 Gt and decrease rapidly thereafter.12 Although agriculture is likely to generate less than 2 percent of global GDP, it alone would fill about 70 percent of the allowable “emissions budget” in 2050 (15 of 21 Gt), leaving almost no space for emissions from other economic sectors and making achievement of even the 2°C target impossible (Figure 3).
Reflecting this dilemma, we define a GHG mitigation gap of 11 Gt: the difference between the 15 Gt of likely annual emissions in 2050 and a target of 4 Gt. The gap represents a nearly 75 percent reduction from the projected level—a reduction in line with the principle of “equal sharing” required from all sources to keep global warming to well below 2°C.
To limit warming to 1.5°C (2.7 degrees Fahrenheit), typical scenarios contemplate similar levels of emissions from agricultural production but require extensive reforestation to offset other emissions. We therefore also explore options for liberating agricultural land to provide such offsets.
Figure 3
Agricultural emissions are likely to be ~70 percent of total allowable emissions for all sectors by 2050, creating an 11 gigaton mitigation gap
Sources
GlobAgri-WRR model, WRI analysis based on IEA (2012); EIA (2012); Houghton (2008); OECD (2012); and UNEP (2013).
A Menu of Solutions
To close these three gaps, we develop a “menu for a sustainable food future”—a menu of actions that can meet these challenges if implemented in time, at scale, and with sufficient public and private sector dedication (Table 1). We analyze the potential of the menu items to sustainably close the food, land, and GHG mitigation gaps by 2050. They are organized into five “courses”:
Reduce growth in demand for food and other agricultural products Increase food production without expanding agricultural land Protect and restore natural ecosystems and limit agricultural land-shifting Increase fish supply Reduce GHG emissions from agricultural production A dominant theme of all menu items is the need to increase the efficiency in use of resources, whether through changes in consumption patterns or uses of land, animals, and other agricultural inputs.
In addition to helping close the three gaps, we impose three additional sustainability criteria on the menu items:
- To reduce poverty and hunger, the menu must neither inflate food prices significantly nor deny agricultural opportunities for small and poor farmers, even as they transition to alternative employment as economies develop.
- Because women’s gains in income disproportionately reduce hunger for the entire household, the menu must provide opportunities for women farmers, who contribute the majority of agricultural labor in many countries and whose productivity has been hampered by unequal access to resources.
- To avoid further overuse and pollution of fresh water, the menu must contribute to pollution control, avoid increases in large-scale irrigation, and conserve or make more efficient use of water wherever possible. Agriculture accounts for roughly 70 percent of global fresh water withdrawals and is the primary source of nutrient runoff from farm fields.13
Table 1
The menu for a sustainable food future: five courses
- 1We calculate the food gap measured in crop calories at 56%. The growth in demand for animal-based foods is calculated differently (i.e., growth in demand for all food calories, including animal- and plant-based foods) and estimated at 68%. Overall, we estimate the total food gap at 55%. Because the “crop calorie gap” and the “food gap” are so similar, we use the terms interchangeably in this report.
- 2UNDESA (2017). The figure of 9.8 billion people in 2050 reflects the “medium fertility variant” or medium population growth scenario (as opposed to the low-growth and high-growth scenarios published by the United Nations Department of Economic and Social Affairs).
- 3To project food demands in 2050, we start with a 2012 FAO projection of the diets that the average person in each country will consume in that year (Alexandratos and Bruinsma [2012]). FAO based its projections on economic growth and income trends and culture in different countries. We adjust these FAO projections moderately, adding fish consumption and including enough additional calories in sub-Saharan Africa and South Asia to ensure sufficient nutrition for everyone, after accounting for waste and unequal distribution. More specifically, we adjusted diets to assure food availability of 3,000 kcal per person per day in sub-Saharan Africa and South Asia by proportionately scaling up all food items in the FAO 2050 projections. “Food availability” is food available to consumers excluding postconsumer waste. The total quantity of calories available must be adequate to feed all individuals after accounting first for this food waste and second for the unequal distribution of food, which means that many individuals will consume less than the regional average. We based the 3,000 kcal/person/day on a recognition that once regions reach this level of food availability, they have low levels of food insecurity. Additionally, the United Nations has added more than half a billion people to its medium-level estimate of the global population in 2050 compared to the scenario used by FAO in 2012, so we further adjust 2050 food demands upward to reflect the new estimate of 9.8 billion people.
- 4See, e.g., Holt-Gimenez (2012); Bittman (2013); and Berners-Lee et al. (2018).
- 5Figures exclude Antarctica. FAO (2011a).
- 6Alexandratos and Bruinsma (2012), Table 4.8. FAO data estimate an increase in arable land in use of 220 Mha from 1962 to 2006. According to FAO (2017a), pasture area has increased by 270 Mha since 1962.
- 7GlobAgri-WRR’s estimates of agricultural production emissions in 2050 employ a variety of calculations and assumptions based on our best estimates of trend factors wherever possible, which we describe more fully in Course 5. Some studies include emissions from regular human burning of savannas and grasslands, but we do not because these systems burn naturally on occasion and we consider any increase in emissions due to human efforts too uncertain. GlobAgri-WRR does, however, consider a smaller set of emissions from the burning of crop residues.
- 8This estimate is based on the GlobAgri-WRR model.
- 9See Figure 18 for a more detailed breakdown of production emissions estimated by GlobAgri-WRR. It excludes downstream emissions from the entire food system in processing, retailing, and cooking, which are overwhelmingly from energy use and must be addressed primarily by a broader transformation of the energy sector.
- 10This figure is based on an estimate of 5 Gt of CO2e emissions per year from land-use change in recent years. It attempts to count carbon losses from the conversion of other lands to agriculture, or conversion of grasslands to cropland, the carbon gains from reversion of agricultural land to forest or other uses, and the ongoing losses of carbon due to degradation of peat. Because it is impossible to estimate land-use-change emissions with data from a single year, we do not choose to pinpoint a specific year for these emissions but instead treat them as a typical rate from recent years. In reality, it is not possible to generate a precise estimate of these numbers because it is not possible to track each hectare of land globally and its carbon changes from year to year. There is a large difference between gross and net losses, and assumptions must be made about rates of carbon gain and loss from land-use change. In addition, many of these data are based on national reporting of net changes in forest area, which therefore assume carbon losses only on the net difference in each country where they occur and carbon gains from net gains in forest where that occurs. This calculation cannot capture the real net losses because the losses in areas losing forest are unlikely to be different (and are often higher) than the gains from regenerating forests. In earlier reports in this series, we estimated emissions from land-use change at 5.5 Gt CO2e on the basis of an average from other estimates found in UNEP (2012); FAO (2012a); and Houghton (2008). These estimates included losses from 2000 to 2005, a period for which FAO’s Forest Resources Assessment (FRA) estimated heavy declines in forest. Several more recent papers have reduced estimates of deforestation and therefore emissions. Smith et al. (2014) estimate 3.2 Gt CO2e/year in 2001–10 including deforestation (3.8 Gt CO2e/year), forest degradation and forest management (-1.8 Gt CO2e/year), biomass fires including peatland fires (0.3 Gt CO2e/year), and drained peatlands (0.9 GtCO2e/year). Another paper estimates 3.3 Gt of CO2 equivalent from land-use change in 2011 but does not include drained peatland (Le Quéré et al. 2012). Federici et al. (2015), who based their estimates on FAO’s 2015 FRA, calculated emissions from net deforestation at 2.904 Gt CO2e/year from 2011 to 2015 but also suggested that this figure was likely 30% too low due to failure to count carbon in some forest pools, which would increase the figure to 3.78 Gt CO2e/year. FAO also estimated peatland emissions separately of 0.9 Gt CO2e/year to the IPCC, leading to a recent FAO estimate of 4.7 Gt/year (Federici et al. 2015). Our peatland emissions estimate of 1.1 Gt CO2e/year also includes fire. Federici et al. (2015) also reported a large increase in “forest degradation,” which is due principally to logging and other nonagricultural activities, and which we do not discuss here. For a summary of the uncertainties and methods, see Searchinger et al. (2013).
- 11See Figure 17 for assumptions about changes in baseline emissions from agricultural production, and “The Land Gap” (p. 8) for assumptions about baseline land-use change.
- 12The 2°C scenario roughly corresponds with the scenario RCP 2.6, which is the lowest climate change scenario analyzed by global modeling teams for the 2014 Intergovernmental Panel on Climate Change (IPCC) assessment. That ambitious scenario, which actually relies on negative emissions in the later part of the century, also assumes that emissions of carbon dioxide, nitrous oxide, and methane fall to roughly 21 Gt of CO2e by 2050, which includes reductions of methane by roughly 50%. Authors’ calculations from data presented in van Vuuren (2011), Figure 6. UNEP (2013) puts the figure for stabilization at 22 Gt. Newer modeling has roughly the same levels as summarized in Sanderson et al. (2016) and UNEP (2017). In this modeling, the emissions target is that required to have a greater than two-thirds chance of holding temperatures to the 2°C goal, reflecting the uncertainties of climate sensitivity to higher GHGs. There are scenarios presented in both papers, particularly UNEP (2017), that allow higher emissions in 2050, but they rely even more on negative emissions later in the century. As we consider the likelihood of any large negative emissions to be questionable at best, we focus only on the scenarios allowing emissions of 21–22 Gt CO2e in 2050. This use of a single emissions target ignores many possible patterns of emissions that would each have the same emissions in 2050 based on 100-year global warming potential but which involve different levels of emissions between 2010 and 2050 that might involve different balances of gases (i.e., different shares of carbon dioxide, nitrous oxide, and methane). Under different variations of such scenarios, the emissions allowable in 2050 would vary greatly. This target for total emissions in 2050, then, merely provides a useful benchmark.Another useful analysis in our baseline is agriculture’s share of allowable cumulative emissions of carbon dioxide alone. Because carbon dioxide persists in the atmosphere so long, some models now try to estimate the maximum cumulative emissions of carbon dioxide (from all sectors) that are consistent with a good chance of holding climate warming to the 2°C goal agreed in Paris. One of the first such studies estimated that maximum cumulative emissions of 670 Gt between 2010 and 2050 would give the world a 75% chance of meeting the target (Meinshausen et al. 2009). UN Environment uses average estimates of 1,000 Gt for a two-thirds chance of meeting the target (UNEP 2017). Another recent study estimates that cumulative emissions of 600 Gt between 2010 and 2050 would enable the world to hold temperature rise to somewhere between 1.5 and 2°C (Figueres et al. 2017). Given these global maximum allowable emissions, our baseline estimate of cumulative agricultural production and land-use-change CO2 emissions of roughly 300 Gt (242 Gt from land-use change and peatlands, and 60 Gt from agricultural energy use) would use up 30–50% of the allowable CO2 emissions from all human sources. Using the cumulative emissions approach, this scenario would also leave too little room for the bulk of GHG emissions from energy use by other economic sectors to reach acceptable climate goals.
- 13FAO (2016); Selman and Greenhalgh (2009).